Milk-borne small extracellular vesicles: kinetics and mechanisms of transport, distribution, and elimination
Abstract
Small extracellular vesicles (sEVs) in milk have the qualities desired for delivering therapeutics to diseased tissues. The production of bovine milk sEVs is scalable (1021 annually per cow), and they resist degradation in the gastrointestinal tract. Most cells studied to date internalize milk sEVs by a saturable process that follows Michaelis-Menten kinetics. The bioavailability of oral milk sEVs is approximately 50%. In addition to crossing the intestinal mucosa, milk sEVs also cross barriers such as the placenta and blood-brain barrier, thereby enabling the delivery of therapeutics to hard-to-reach tissues. In time course studies, levels of milk sEVs peaked in the intestinal mucosa, plasma, and urine approximately 6 h and returned to baseline 24 h after oral gavage in mice. In tissues, milk sEV levels peaked 12 h after gavage. Milk sEVs appear to be biologically safe. No cytokine storm was observed when milk sEVs were added to cultures of human peripheral blood mononuclear cells or administered orally to rats. Liver and kidney function and erythropoiesis were not impaired when milk sEVs were administered to rats by oral gavage for up to 15 days. Protocols for loading milk sEVs with therapeutic cargo are available. Currently, the use of milk sEVs (and other nanoparticles) in the delivery of therapeutics is limited by their rapid elimination through internalization by macrophages and lysosomal degradation in target cells. This mini review discusses the current knowledge base of sEV tissue distribution, excretion in feces and urine, internalization by macrophages, and degradation in lysosomes.
Keywords
INTRODUCTION
Small extracellular vesicles (sEVs) in milk have garnered attention for delivering therapeutics to diseased tissues. Most papers referenced in this mini review used exosome-rich preparations of sEVs, as evidenced by sizes that averaged 100 nm, the detection of exosome marker proteins, and the low abundance of markers of microsomes and nanoparticles such as lipoproteins, fat globules, and casein micelles[1-3]. It is safe to suggest that all preparations were contaminated with sEVs other than exosomes to some extent[4]. The National Nanotechnology Initiative defines nanoparticles as particles no larger than 100 nm in diameter, which might be relevant when using milk sEVs (“exosomes”) in nanomedicine[5]. Milk sEVs hold great promise in nanomedicine based on the following qualities. The production of milk sEVs is scalable, e.g., bovine milk contains approximately 1014 sEVs per mL, and cows produce approximately 10,800 kg of milk annually[6,7]. Milk sEVs protect their cargo against degradation in the gastrointestinal tract and industrial processing. Cells internalize milk sEVs by endocytosis, which confers a pathway for the delivery of therapeutic cargo that otherwise might not easily cross cell membranes[8-11]. Protocols have been reported for loading sEVs, including those from milk, with therapeutic cargos, including drugs, small RNAs, and proteins[12-16]. Milk sEVs are absorbed following oral administration and accumulate primarily in the intestinal mucosa, brain, spleen, and liver[13,17,18]. Milk sEVs do not elicit detectable immune reactions in humans and mice and do not compromise erythropoiesis, liver and kidney function, food and water intake, and body weight gain in rats and mice[18-20]. Milk sEVs cross barriers such as the placenta and blood-brain barrier and elicit changes in gene expression[21,22].
The qualities discussed above have triggered an interest in utilizing milk sEVs for delivering therapeutics and diagnostics to hard-to-reach diseased tissues and tumors. Initial studies yielded promising results. For example, when bovine milk sEVs were loaded with lung cancer KRASG12S-specific siRNA and delivered to athymic nude mice by intravenous injection, the volume of A549 lung cancer xenografts was 54% smaller compared to the vehicle control[14]. Results were similar when drug-loaded bovine milk sEVs were administered orally. For example, when bovine milk sEVs were loaded with paclitaxel and administered to female athymic nude mice by oral gavage, the growth of A549 lung cancer xenografts was decreased by
There are limitations to using milk sEVs in drug delivery, including their rapid elimination from body fluids. This mini review discusses the current knowledge base on milk sEV elimination in feces and urine, elimination by macrophages, and degradation in lysosomes. The discussion is framed in the context of milk sEV distribution among tissues and cell compartments. While recent reviews have discussed the roles of milk sEVs in nutrition and drug delivery, this mini review is unique because of its focus on pharmacokinetics, including the kinetics of uptake and elimination[24-28].
KINETICS OF MILK SEV INTERNALIZATION IN CELL CULTURES
Most cells studied to date internalize milk sEVs through a saturable process that follows Michaelis-Menten kinetics. Maximal rate of transport (Vmax) and Michaelis constant (Km) have been reported for bovine milk sEVs internalization in mouse brain endothelial bEnd.3 cells, mouse BV2 microglia, human colon carcinoma Caco-2 cells, rat small intestinal IEC-6 cells, and human umbilical cord HUVEC cells are summarized in Table 1[17,22,29]. Non-transformed human intestinal epithelial crypt-like HIEC cells appear to internalize human milk sEVs, but transport kinetics were not assessed[30]. Many of these studies used lipophilic dyes for labeling sEVs. Lipophilic dyes may detach from sEV membranes and transfer to other lipophilic particles and compounds, which is a potential limitation of these reports[31]. Studies in cell cultures cannot replace bioavailability studies in humans and animal models, but they can provide important mechanistic insights into sEV internalization and transport across layers of recipient cells[32]. For example, Z-stack microscopy was used to demonstrate that bEnd.3 cells internalized sEVs using labeled RNA as the tracer, as opposed to the sEVs adsorbing to the cell surface[22]. Dual chamber systems were used to demonstrate that milk sEVs monolayers are transported across monolayers of bEnd.3 and Caco-2 cultures[17,22]. Reporter plasmid studies provided experimental evidence that human peripheral blood mononuclear cells internalized unlabeled milk sEVs[33]. It is unknown what constitutes a level of natural, unloaded milk sEVs that elicits a biological effect in recipient cells.
Cell | Vmax | Unit | Km | Unit |
bEnd.3 | 0.77 ± 0.18 | ng sEV protein × 19,000 cells-1 × 45 min-1 | 1.8 ± 2.0 | 1011 sEV protein × mL media-1 |
BV2 | 0.66 ± 0.14 | ng sEV protein × 19,000 cells-1 × 45 min-1 | 1.9 ± 1.9 | 1011 sEV protein × mL media-1 |
Caco-2 | 0.083 ± 0.057 | ng sEV protein × 81,750 cells-1 × h-1 | 55.5 ± 48.6 | μg sEV protein × 200 μL media-1 |
IEC-6 | 0.14 ± 0.01 | ng sEV protein × 36,375 cells-1 × h-1 | 152 ± 39.5 | μg sEV protein × 200 μL media-1 |
HUVEC | 0.057 ± 0.004 | ng sEV protein × 40,000 cells-1 × h-1 | 17.97 ± 3.84 | μg sEV protein × 200 μL media-1 |
TIME COURSES OF MILK SEVS IN FECES, BODY FLUIDS, AND TISSUES FOLLOWING ORAL ADMINISTRATION
Data are scarce regarding the fecal and urinary excretion of milk sEVs. When bovine milk sEVs were labeled with a carbonyl-reactive fluorescent dye, HiLyte-750TM, and administered by oral gavage, approximately 50% of the dose was recovered in feces, and excretion was complete within 24 h[34]. Incubation of HiLyte-labeled milk sEVs in artificial intestinal fluid provided experimental evidence that the label was not released and, therefore, sEVs (as opposed to free dye) were traced. In time course studies, levels of milk sEVs peaked in the intestinal mucosa, plasma, and urine approximately 6 h and returned to baseline 24 h after oral gavage in mice. In tissues, milk sEV levels peaked 12 h after gavage. It is possible that some of the fecal sEVs have gone through enterohepatic circulation, i.e., were excreted through bile into the gut after absorption, like the enterohepatic circulation of cholesterol and bile acids[35]. Similarly, it is possible that milk sEVs were released into the intestinal lumen by the shedding of intestinal cells. Approximately 2 × 108 cells are shed from the small intestine per day in mice[36].
The acidic environment in the stomach might cause changes in sEV morphology and partial degradation of the tetraspanins CD9 and CD81[37]. The implications for intestinal absorption of milk sEVs are unknown. Likewise, it is unknown whether changes in milk sEV size and morphology, caused by industrial processing, have an effect on the bioavailability and disposition[38].
When bovine milk sEVs were loaded with fluorophore (IRDye)-labeled RNA and administered by oral gavage, strong fluorescence was observed in the kidney 6 h after administration in mice[13]. This is consistent with the time courses of urinary excretion of HiLyte-750TM-labeled bovine milk sEVs in mice, which peaked 6 h after oral gavage[34].
Dose-response time-course studies of the pharmacokinetics of human embryonic kidney Expi293F cell-derived sEVs in macaques (Macaca nemestrina) showed that the plasma half-life decreased from approximately 40 min for the low doses tested to 11 min for the highest dose tested[39]. The authors did not report the number of biological repeats, and it is unlikely that the pharmacokinetics are the same for kidney cell-derived sEVs and milk sEVs.
ELIMINATION BY MACROPHAGES
Macrophages play an important role in the clearance of foreign sEVs, including milk sEVs[11,40-42]. Milk sEV distribution studies provided circumstantial evidence for the role of macrophages in milk sEV elimination by demonstrating that bovine milk sEVs accumulated in large quantities in macrophage-rich tissues such as spleen and liver in addition to the brain [Figure 1A][13,18]. The liver was also a major accumulation site, in addition to intestinal mucosa and brain, when milk sEVs were obtained from the same species, i.e., when neonate mice were nursed by a dam that secreted milk sEVs endogenously labeled with a fluorescent fusion protein [Figure 1B][22]. The fusion protein studies provide compelling evidence that milk sEVs, not detached labels, were traced. Dual chamber protocols, Z-stack confocal microscopy, and serial two-photon tomography were used to demonstrate that milk sEVs cross the intestinal and blood-brain barriers and are internalized by target cells[17,22]. The uptake of bovine milk sEVs was formally assessed in murine bone marrow-derived macrophages and revealed that Class A scavenger receptor 1/2 is the primary transporter facilitating sEV internalization by macrophages[11]. Receptor knockout decreased sEV uptake by approximately 60%. Transporter capacity in macrophages exceeded capacities observed in intestinal, vascular, and brain endothelial cells[17,22,29]. When murine melanoma-derived sEVs were administered to macrophage-depleted mice, the mean residence time in plasma and clearance were 5.2-fold and 63-fold, greater and smaller, respectively, compared to control mice [Table 2][42]. It remains to be determined if the effects are similar for milk sEVs. Experimental evidence suggests that milk sEVs transport oligosaccharides into macrophages and modulate immunity and attenuate Escherichia coli infection in mice[43].
Figure 1. Tissue distribution of milk sEVs in mice. (A) Distribution of bovine milk sEVs loaded with fluorophore (ATTO)-labeled miR-375 in Balb/c mice. Modified from Manca et al. with permission from the original publisher, Nature Springer[13]. To view a copy of this license, visit http://creativecommons.org/licenses/by/4.0/; (B) Accumulation of enhanced green fluorescence protein (eGFP)-positive milk sEVs in peripheral tissues and the small intestinal mucosa in wild-type (WT) pups fostered to exosome and cargo tracking (ECT) dams and nursed for 17 days. ECT mice secrete sEVs labeled with an eGFP fusion protein in milk. WT pups fostered to WT dams served as controls. From Zhou et al. with permission from the original publisher, Frontiers Media SA[22]. To view a copy of this license, visit http://creativecommons.org/licenses/by/4.0/.
Elimination kinetics of sEVs from murine melanoma B16BL6 cells in macrophage-depleted and control Balb/c mice. Macrophages were depleted by treating mice with clodronate
Mice | sEV dose (μg) | MRT (h) | CL (mL/h) |
Depleted | 5.0 | 3.6 ± 0.7* | 0.65 ± 0.16* |
Control | 5.0 | 0.69 ± 0.09 | 41.2 ± 6.8 |
DEGRADATION IN LYSOSOMES AND ESCAPE PATHWAYS
Intracellular bovine milk sEVs accumulated in cytoplasm and cytoplasmic organelles (presumably lysosomes and multivesicular body), whereas nuclear localization was not apparent in murine bone marrow-derived macrophages[11]. The biogenesis of sEVs occurs in the endosomal system, including multivesicular bodies[44]. sEVs and their cargos have three metabolic fates [Figure 2]: delivery to lysosomes for degradation, retrofusion to the limiting membrane of the multivesicular body and subsequent release of cargo into the cytoplasm, and fusion with the plasma membrane for subsequent release into the extracellular space[45,46]. A small fraction of sEVs escape lysosomal degradation, e.g., only approximately 17% of sEVs in human melanoma MelJuSo cells fused back to the limiting membrane of the multivesicular body[46]. No reports are available regarding the percentage of milk sEVs released into the extracellular space by fusion with the plasma membrane.
CONCLUSION
Milk sEVs have properties conducive to their use in drug delivery, including, but not limited to, the transfer across the blood-brain barrier. A substantial fraction of milk sEVs is cleared by macrophages and presumably degraded in lysosomes in target cells. It is desirable to devise technologies that lower macrophage uptake and lysosomal degradation of milk sEVs to realize their full potential in the delivery of therapeutic agents.
DECLARATIONS
Authors’ contributionsConceived the idea for the review: Zempleni J
Searched the literature and wrote the manuscript: Munir J, Zempleni J
Generated the figures: Ngu A, Zempleni J
Edited the final version of the manuscript: Zempleni J
Availability of data and materialsNot applicable.
Financial support and sponsorshipThis work was supported by the National Institutes of Health (grant numbers P20 GM104320 and R21 OD028749); the National Institute of Food and Agriculture (grant numbers 2020-67017-30834 and 2022-67021-36407); the SynGAP Research Fund, and the United States Department of Agriculture (grant numbers W-4002 and Hatch NEB-36-096).
Conflicts of interestThe authors declare no conflicts of interest. J. Zempleni serves as a consultant for PureTech Health, Inc. (Boston, MA) and on the Editorial Board of Extracellular Vesicles and Circulating Nucleic Acids.
Ethical approval and consent to participateNot applicable.
Consent for publicationNot applicable.
Copyright© The Author(s) 2023.
REFERENCES
1. György B, Szabó TG, Pásztói M, et al. Membrane vesicles, current state-of-the-art: emerging role of extracellular vesicles. Cell Mol Life Sci 2011;68:2667-88.
2. Zonneveld MI, Brisson AR, van Herwijnen MJ, et al. Recovery of extracellular vesicles from human breast milk is influenced by sample collection and vesicle isolation procedures. J Extracell Vesicles 2014;3:24215.
3. Théry C, Witwer KW, Aikawa E, et al. Minimal information for studies of extracellular vesicles 2018 (MISEV2018): a position statement of the International Society for Extracellular Vesicles and update of the MISEV2014 guidelines. J Extracell Vesicles 2018;7:1535750.
4. Leiferman A, Shu J, Upadhyaya B, Cui J, Zempleni J. Storage of extracellular vesicles in human milk, and microrna profiles in human milk exosomes and infant formulas. J Pediatr Gastroenterol Nutr 2019;69:235-8.
5. National Nanotechnology Institute. Nantional nanotechnology initiative. Alexandria, VA: National Nanotechnology Coordination Office; 2022. Available from: www.nano.gov [Last accessed on 11 Jul 2023].
6. Sukreet S, Braga CP, An TT, et al. Isolation of extracellular vesicles from byproducts of cheesemaking by tangential flow filtration yields heterogeneous fractions of nanoparticles. J Dairy Sci 2021;104:9478-93.
7. Statista, Inc. Milk produced per cow in the United States from 1999 to 2023 (in pounds). Available from: https://www.statista.com/statistics/194935/quantity-of-milk-produced-per-cow-in-the-us-since-1999/ [Last accessed on 11 Jul 2023].
8. Izumi H, Kosaka N, Shimizu T, Sekine K, Ochiya T, Takase M. Bovine milk contains microRNA and messenger RNA that are stable under degradative conditions. J Dairy Sci 2012;95:4831-41.
9. Benmoussa A, Lee CH, Laffont B, et al. Commercial dairy cow milk microRNAs resist digestion under simulated gastrointestinal tract conditions. J Nutr 2016;146:2206-15.
10. Howard KM, Jati Kusuma R, Baier SR, et al. Loss of miRNAs during processing and storage of cow's (Bos taurus) milk. J Agric Food Chem 2015;63:588-92.
11. Khanam A, Yu J, Zempleni J. Class A scavenger receptor-1/2 facilitates the uptake of bovine milk exosomes in murine bone marrow-derived macrophages and C57BL/6J mice. Am J Physiol Cell Physiol 2021;321:C607-14.
12. Agrawal AK, Aqil F, Jeyabalan J, et al. Milk-derived exosomes for oral delivery of paclitaxel. Nanomedicine 2017;13:1627-36.
13. Manca S, Upadhyaya B, Mutai E, et al. Milk exosomes are bioavailable and distinct microRNA cargos have unique tissue distribution patterns. Sci Rep 2018;8:11321.
14. Aqil F, Munagala R, Jeyabalan J, et al. Milk exosomes-natural nanoparticles for siRNA delivery. Cancer Lett 2019;449:186-95.
15. Sterzenbach U, Putz U, Low LH, Silke J, Tan SS, Howitt J. Engineered exosomes as vehicles for biologically active proteins. Mol Ther 2017;25:1269-78.
16. Ogunnaike M, Wang H, Zempleni J. Bovine mammary alveolar MAC-T cells afford a tool for studies of bovine milk exosomes in drug delivery. Int J Pharm 2021;610:121263.
17. Wolf T, Baier SR, Zempleni J. The intestinal transport of bovine milk exosomes is mediated by endocytosis in human colon carcinoma Caco-2 cells and rat small intestinal IEC-6 cells. J Nutr 2015;145:2201-6.
18. Munagala R, Aqil F, Jeyabalan J, Gupta RC. Bovine milk-derived exosomes for drug delivery. Cancer Lett 2016;371:48-61.
19. Mutai E, Ramer-tait AE, Zempleni J. MicroRNAs in bovine milk exosomes are bioavailable in humans but do not elicit a robust pro-inflammatory cytokine response. ExRNA 2020;2:1-9.
20. Leiferman A, Shu J, Grove R, Cui J, Adamec J, Zempleni J. A diet defined by its content of bovine milk exosomes and their RNA cargos has moderate effects on gene expression, amino acid profiles and grip strength in skeletal muscle in C57BL/6 mice. J Nutr Biochem 2018;59:123-8.
21. Sadri M, Shu J, Kachman SD, Cui J, Zempleni J. Milk exosomes and miRNA cross the placenta and promote embryo survival in mice. Reproduction 2020;160:501-9.
22. Zhou F, Ebea P, Mutai E, et al. Small extracellular vesicles in milk cross the blood-brain barrier in murine cerebral cortex endothelial cells and promote dendritic complexity in the hippocampus and brain function in C57BL/6J mice. Front Nutr 2022;9:838543.
23. Thepharmaletter. PureTech out-licenses milk exosomes technology in $1 billion deal. Available from: https://www.thepharmaletter.com/article/puretech-out-licenses-milk-exosomes-technology-in-1-billion-deal. [Last accessed on 11 Jul 2023].
24. Adriano B, Cotto NM, Chauhan N, Jaggi M, Chauhan SC, Yallapu MM. Milk exosomes: Nature's abundant nanoplatform for theranostic applications. Bioact Mater 2021;6:2479-90.
25. Cieślik M, Nazimek K, Bryniarski K. Extracellular vesicles-oral therapeutics of the future. Int J Mol Sci 2022;23:7554.
26. Chutipongtanate S, Morrow AL, Newburg DS. Human milk extracellular vesicles: a biological system with clinical implications. Cells 2022;11:2345.
27. Ngu A, Wang S, Wang H, Khanam A, Zempleni J. Milk exosomes in nutrition and drug delivery. Am J Physiol Cell Physiol 2022;322:C865-74.
28. Tian MY, Hao DX, Liu Y, et al. Milk exosomes: an oral drug delivery system with great application potential. Food Funct 2023;14:1320-37.
29. Kusuma RJ, Manca S, Friemel T, Sukreet S, Nguyen C, Zempleni J. Human vascular endothelial cells transport foreign exosomes from cow's milk by endocytosis. Am J Physiol Cell Physiol 2016;310:C800-7.
30. Kahn S, Liao Y, Du X, Xu W, Li J, Lönnerdal B. Exosomal micrornas in milk from mothers delivering preterm infants survive
31. Takov K, Yellon DM, Davidson SM. Confounding factors in vesicle uptake studies using fluorescent lipophilic membrane dyes. J Extracell Vesicles 2017;6:1388731.
32. Roerig J, Schiller L, Kalwa H, et al. A focus on critical aspects of uptake and transport of milk-derived extracellular vesicles across the Caco-2 intestinal barrier model. Eur J Pharm Biopharm 2021;166:61-74.
33. Baier SR, Nguyen C, Xie F, Wood JR, Zempleni J. MicroRNAs are absorbed in biologically meaningful amounts from nutritionally relevant doses of cow milk and affect gene expression in peripheral blood mononuclear cells, HEK-293 kidney cell cultures, and mouse livers. J Nutr 2014;144:1495-500.
34. Khanam A, Ngu A, Zempleni J. Bioavailability of orally administered small extracellular vesicles from bovine milk in C57BL/6J mice. Int J Pharm 2023;639:122974.
35. van de Peppel IP, Verkade HJ, Jonker JW. Metabolic consequences of ileal interruption of the enterohepatic circulation of bile acids. Am J Physiol-Gastr L 2020;319:G619-25.
36. Williams JM, Duckworth CA, Burkitt MD, Watson AJ, Campbell BJ, Pritchard DM. Epithelial cell shedding and barrier function: a matter of life and death at the small intestinal villus tip. Vet Pathol 2015;52:445-55.
37. Rahman MM, Shimizu K, Yamauchi M, et al. Acidification effects on isolation of extracellular vesicles from bovine milk. PLoS One 2019;14:e0222613.
38. Kleinjan M, van Herwijnen MJ, Libregts SF, van Neerven RJ, Feitsma AL, Wauben MH. Regular industrial processing of bovine milk impacts the integrity and molecular composition of extracellular vesicles. J Nutr 2021;151:1416-25.
39. Driedonks T, Jiang L, Carlson B, et al. Pharmacokinetics and biodistribution of extracellular vesicles administered intravenously and intranasally to Macaca nemestrina. J Extracell Biol 2022;1:e59.
40. Wiklander OP, Nordin JZ, O'Loughlin A, et al. Extracellular vesicle
41. Izumi H, Tsuda M, Sato Y, et al. Bovine milk exosomes contain microRNA and mRNA and are taken up by human macrophages. J Dairy Sci 2015;98:2920-33.
42. Imai T, Takahashi Y, Nishikawa M, et al. Macrophage-dependent clearance of systemically administered B16BL6-derived exosomes from the blood circulation in mice. J Extracell Vesicles 2015;4:26238.
43. He Y, He Z, Leone S, Liu S. Milk exosomes transfer oligosaccharides into macrophages to modulate immunity and attenuate Adherent-Invasive E. coli (AIEC) Infection. Nutrients 2021;13:3198.
44. Woodman PG, Futter CE. Multivesicular bodies: co-ordinated progression to maturity. Curr Opin Cell Biol 2008;20:408-14.
45. Joshi BS, de Beer MA, Giepmans BNG, Zuhorn IS. Endocytosis of extracellular vesicles and release of their cargo from endosomes. ACS Nano 2020;14:4444-55.
Cite This Article
Export citation file: BibTeX | RIS
OAE Style
Ngu A, Munir J, Zempleni J. Milk-borne small extracellular vesicles: kinetics and mechanisms of transport, distribution, and elimination. Extracell Vesicles Circ Nucleic Acids 2023;4:339-46. http://dx.doi.org/10.20517/evcna.2023.25
AMA Style
Ngu A, Munir J, Zempleni J. Milk-borne small extracellular vesicles: kinetics and mechanisms of transport, distribution, and elimination. Extracellular Vesicles and Circulating Nucleic Acids. 2023; 4(3): 339-46. http://dx.doi.org/10.20517/evcna.2023.25
Chicago/Turabian Style
Ngu, Alice, Javaria Munir, Janos Zempleni. 2023. "Milk-borne small extracellular vesicles: kinetics and mechanisms of transport, distribution, and elimination" Extracellular Vesicles and Circulating Nucleic Acids. 4, no.3: 339-46. http://dx.doi.org/10.20517/evcna.2023.25
ACS Style
Ngu, A.; Munir J.; Zempleni J. Milk-borne small extracellular vesicles: kinetics and mechanisms of transport, distribution, and elimination. Extracell. Vesicles. Circ. Nucleic. Acids. 2023, 4, 339-46. http://dx.doi.org/10.20517/evcna.2023.25
About This Article
Copyright
Data & Comments
Data
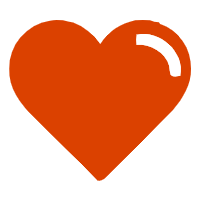

Comments
Comments must be written in English. Spam, offensive content, impersonation, and private information will not be permitted. If any comment is reported and identified as inappropriate content by OAE staff, the comment will be removed without notice. If you have any queries or need any help, please contact us at support@oaepublish.com.